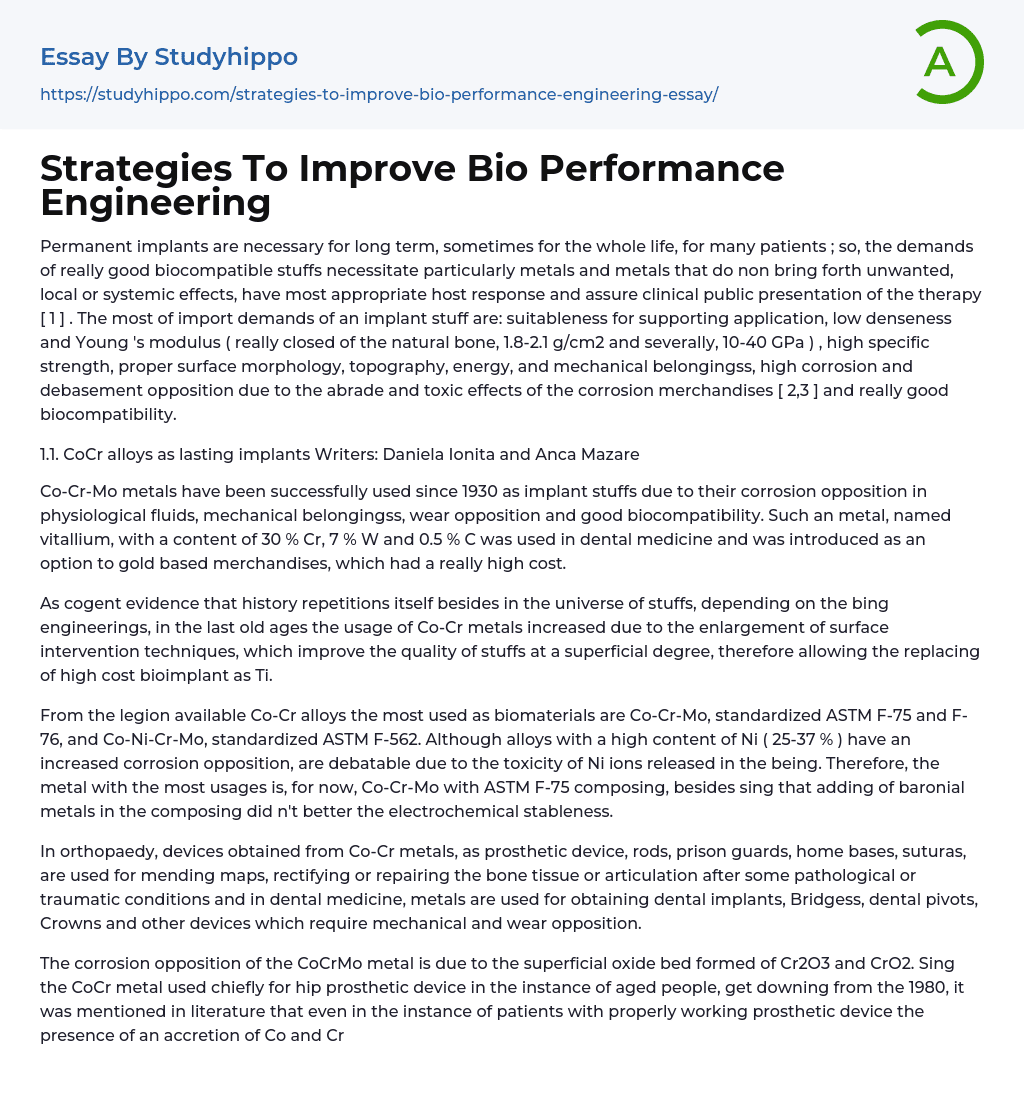
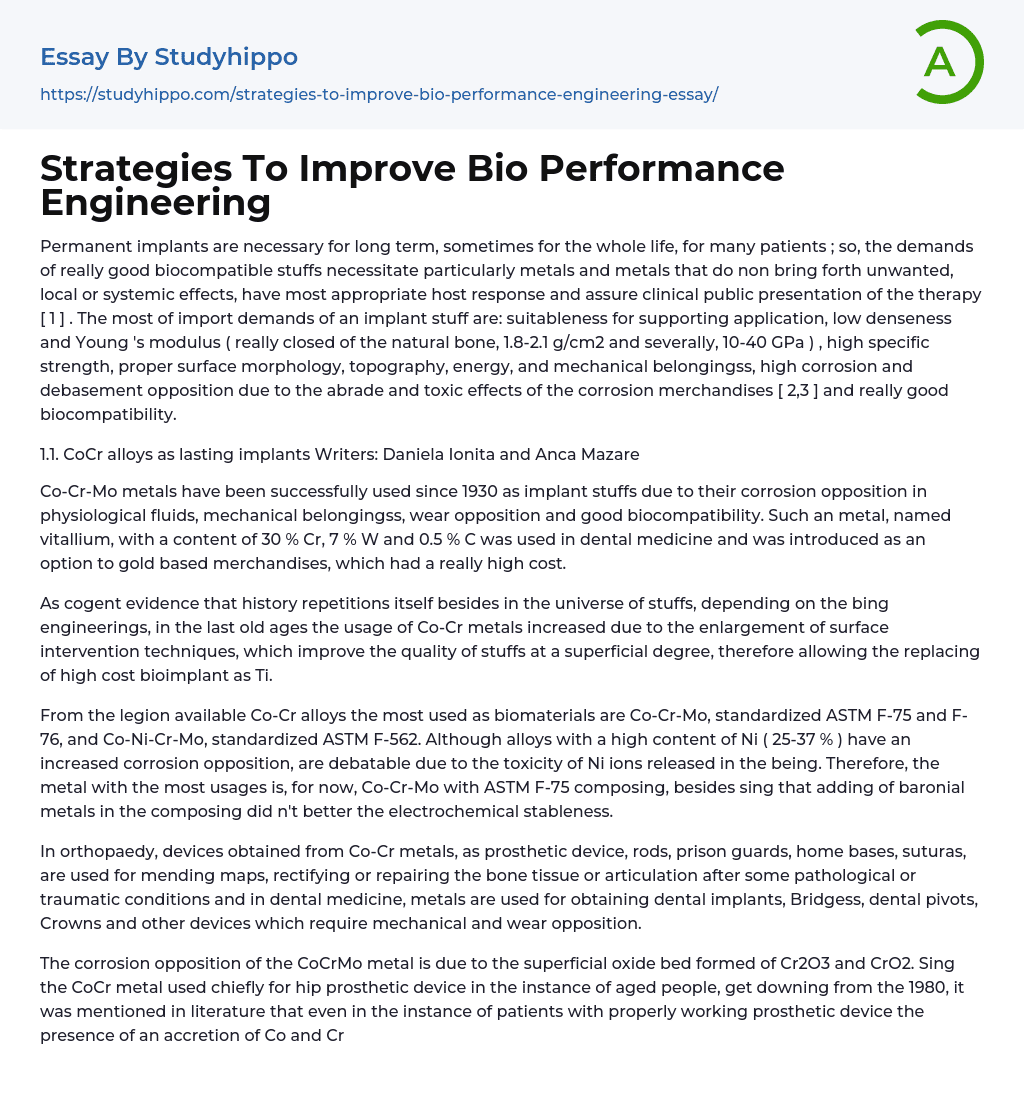
Strategies To Improve Bio Performance Engineering Essay Example
The demand for biocompatible materials that do not produce unwanted effects is crucial for patients who require permanent implants. Metals, specifically Co-Cr alloys like vitallium, are preferred due to their ability to meet these requirements and ensure successful clinical outcomes. These alloys have been used as implant materials since 1930 and offer corrosion resistance in bodily fluids, mechanical properties, wear resistance, and biocompatibility. Advancements in surface treatment techniques have further increased the use of Co-Cr metals in recent years, allowing for the replacement of expensive bioimplants like Titanium. Among the available Co-Cr alloys, Co-Cr-Mo (ASTM F-75 and F-76) and Co-Ni-Cr-Mo (ASTM F-562) are commonly used as biomaterials [1][1.1] (Writers: Daniela Ionita and Anca Mazare).However, alloys containing a high amount of Nickel (25-37%) pose challenges due to the toxicity of released Nickel ions. Currently, t
...he most commonly used metal is Co-Cr-Mo with ASTM F-75 composition as noble metals did not enhance electrochemical stability. In orthopedics, Co-Cr metals such as prostheses, rods, plates, sutures are utilized for healing and correcting bone tissue or joints after various pathological or traumatic conditions. In dentistry, metals like dental implants, bridges, dental pivots, crowns are employed for their mechanical strength and wear resistance. The corrosion resistance of CoCrMo metal is attributed to the formation of a superficial oxide layer consisting of Cr2O3 and CrO2. However, literature reveals that CoCr metal used primarily in elderly individuals for hip prosthetic devices since the 1980s can result in elevated levels of Co and Cr ions in vital organs like the liver, kidneys, and spleen even when prosthetic devices function properly. This release of ions from implant material accumulation can cause tissue inflammation leading t
implant failure ultimately. Biomedical materials must possess specific properties with biocompatibility being crucial for implants followed by corrosion resistance. Compared to other metals in physiological fluids; however; CoCr alloy devices exhibit lower corrosion resistance which limits their use only for temporary implants.
Recent research has explored the use of surface treatments on stainless steel as a cost-effective alternative for long-lasting implants. These treatments aim to improve biocompatibility, bone integration, wear and corrosion resistance, prevent release of toxic substances, and blood clot formation.
One approach is through surface nano-functionalization engineerings. Research indicates that modifying the surface properties of biocompatible materials using chemical methods can partially control crucial biological processes such as protein assimilation, cell growth, and gene expression. This can be achieved by direct chemical alterations with acids and oxidizers or deposition of bioactive and antimicrobial layers.
Chemical interventions involving acids, bases, and hydrogen peroxide have been used for surface modification at the nanoscale level. These chemical reactions between materials and solutions create textured surfaces at micrometer and submicrometer scales. Studies have shown that chemically treated surfaces enhance adhesion and proliferation of osteogenic cells. In our case, we utilized 10mm diameter Co-Cr metal samples measuring 2mm in height.A Co-Cr-Mo metal was used, which had a composition similar to ASTM F75 metal. The samples were ground with silicon carbide papers of varying grit sizes, washed with deionised water and ethyl alcohol, dried, and stored in a desiccator. Before testing, the samples were treated with 20% nitric acid for 30 minutes at room temperature according to ASTM F86 standard. This treatment increased the thickness of the surface oxide layer and improved the corrosion resistance properties of the film. Atomic force microscopy
(AFM) analysis showed an average roughness of 0.186 ?m ± 0.10 mm on the chemically treated CoCrMo alloys. To promote direct bonding with bone, bioactive layers like hydroxyapatite or bioactive glass can be deposited using a biomimetic process. This involves chemical treatment in an alkaline solution followed by heat treatment and immersion in simulated body fluid (SBF). Immersion in SBF serves as an initial assessment of a biocompatible material's bioactivity. After mechanical processing, the metallic samples were immersed in a 5M NaOH solution at 60°C for 24 hours.After undergoing an alkaline treatment, the samples were rinsed and dried. SEM analysis revealed a porous surface (Fig.1), while EDS indicated the presence of sodium compounds on the CoCrMo sample surface, formed during thermal treatment (Fig.1). The images show the CoCrMo metal treated in 5M NaOH. Following this, the samples were immersed in SBF for a total of 21 years at 37oC. SEM analysis showed a thin layer of amorphous Ca phosphate on the CoCrMo sample surface upon submergence. This layer acts as a precursor for crystalline hydroxyapatite formation. Fig.2 displays both the SEM image and EDS spectrum depicting hydroxyapatite covering the CoCrMo sample surfaces. Deposition of hydroxyapatite significantly alters properties of coated CoCrMo surfaces.SEM analysis demonstrated a porous bed with approximately 5Aµm pore dimensions and three times greater roughness than grounded surfaces (0.624 nanometer). Hydroxyapatite can be deposited on CoCrMo metal using chemical or electrochemical methods, as well as laser deposition methodsElectrochemical deposition has the advantage of creating hydroxyapatite coatings composed of nanometric crystals, which promote bone remodeling and maturation. Researchers have studied various factors that affect the electrolytic process. These factors include the composition of the
electrolyte [17], electrolyte temperature [18], current density [19], composition of the substrate metal, hydrothermal treatment after electrochemical synthesis [20], and hydrothermal-electrochemical conditions that impact different aspects of the interface. These aspects include sedimentation amounts, size, shape, morphology, microstructure of the HA sedimentation, and bonding strength of the HA-metallic substrate interface.
To obtain an n-Ag coating on a CoCrMo substrate, after mechanical processing, silanization was performed on metallic electrodes for 1.5 hours in APS (3-aminopropyltrietoxisilane). The electrodes were then washed with acetone and distilled water and dried at 25oC. Subsequently, they were incubated for 3 hours in a solution containing 20 mL of 0.25mM AgNO3, 25 mL of 0.25mM trisodium citrate, and 0.6 mL of 10mM NaBH4 under vigorous stirring [21].
The topography analysis of coated surfaces was conducted using AFM technique with scanned areas measuring 20 x 20 µm in contact mode. Fig.3 presents the 3D AFM image showing the deposition of n-Ag film on CoCrMo samples.The deposition of n-Ag on CoCrMo metals is revealed in an AFM image, showing a dendritic nature and a high mean square roughness value (758 nm) [Fig.3]. The presence of n-Ag increases the roughness (Rq) from 451 to 545 nanometers. Both a recent review paper by Mendonca et al.[22] and clinical studies [23-25] suggest that surface modification is an effective strategy for enhancing the biological functionalities of metallic implants for in vivo clinical applications.
B. Corrosion in the body
Biocompatibility is crucial for biomaterials, as shown in Fig.4 with several factors influencing it. Among these factors, corrosion plays a significant role. Metallic materials are corroded by physiological solutions, which can have various detrimental effects when used as implants: (I) release of non-biocompatible
metal ions/corrosion products, (II) shortened lifespan of the implant device leading to additional surgeries, and (III) reduced human life expectancy. Electrochemical methods used for analyzing corrosion processes can be categorized into two types.The text discusses the use of corrosion measurements, such as Open Circuit Potential (OCP) and Electrochemical Impedance Spectroscopy (EIS), to evaluate localized corrosion in corrosion-resistant materials. These measurements are used to monitor the degradation of implants in various fluids, including artificial body fluid (SBF), Hank's solution, Ringer's solution, and artificial saliva. The pH level of bodily fluids typically ranges from 7.35 to 7.45 but may decrease to 5.2 during implantation in hard tissues before returning to normal within two weeks at a temperature of approximately 37°C.
Numerous studies have investigated the surface oxide chemistry of Co-Cr metal under different conditions. Exposed samples tend to shift towards more active potentials until reaching a stable state, while covered samples experience a shift towards more positively charged potentials and reach equilibrium quickly. Samples coated with hydroxyapatite achieve equilibrium the fastest, indicating the presence of a stable film on the surfaces of CoCrMo metals.
Table 1 presents the potential values after 60 minutes for CoCrMo electrodes with various surface interventions in physiological media.An increase in the Open Circuit Potential (OCP) indicates stable coating/insulation behavior. The electrode values for the simulated body fluid (SBF) solution are more positively charged compared to the Ringer solution, attributed to the composition of SBF which deposits carbonates and phosphates on the electrode surface over time [32] [33]. Observations on local corrosion were obtained through potentiodynamic polarization and linear polarization methods. Potentiodynamic polarization involved plotting cyclic polarization curves using a Voltalab 40 PGZ 301 potentiostat/galvanostat
and computer software VoltaMaster 4. Both the anodal polarization curve and hysteresis curve were followed [32] [33]. The curves were plotted starting at -800 mV and ending at 1200 mV with a sweep rate of 2 mV/s. These curves helped identify key electrochemical parameters related to corrosion and passivation processes: corrosion potential (Ecorr), corrosion critical current (Icorr), corrosion rate (Vcorr), and passivation sphere. A Pt electrode served as the auxiliary electrode, and an Ag/AgCl electrode immersed in a concentrated solution of KCl served as the reference electrode. The linear polarization technique was used to determine the polarization resistance (Rp), corrosion current densities (icorr), corrosion rates (Vcorr), and ion release rates. A scan rate of 10 mV/s was applied within a range of ±50 mV from the open circuit potential using Voltalab 40 equipment.The software Voltamaster 4 directly provides values for Rp, icorr, and Vcorr. The prevailing redox conditions are crucial from a corrosion perspective, and the oxygen content in the environment can vary depending on the specific application. For metals that rely on a Cr2O3-rich passive film on their surfaces, highly oxidizing conditions can dissolve this film by forming soluble Cr (VI) species. In biological reactions, more reactive oxygen species like H2O2 can be generated along with dissolved molecular oxygen (O2). The stability of the inactive movie depends on O availability. Fig.5 displays Tafel secret plans for CoCrMo uncovered and coated with HA in an SBF solution, which were used to determine electrochemical parameters listed in Table 2. Cyclic voltammetry trials (Fig.6) show that coatings improve the electrochemical stability of biomaterials in physiological fluids. There is no active/passive transition and the inactive range is determined
immediately after the Tafel region. The inactive range extends from -/0.3 V to about 0.4 V for uncoated samples or 0.6 V for CoCrMo/HA before being interrupted by an increase in current density. Potentiodynamic curve exhibits a peak at potentials higher than +0.8 V (SCE), attributed to transpassive oxidation of Cr3+ to Cr6+ according to [34].The reaction is as follows: 3Cr3+ + 4H2O -> Cr2O3 + 6H+ + 6e-. Cyclic voltammetry trials in the field of metallic biomaterials have demonstrated that nanoscale surface modification can enhance bioactive capacity. However, many of the described modification approaches still need to undergo in vivo testing. Various types of coatings have been utilized as surface alterations to decrease corrosion rate [35]. Furthermore, ion plating or ion nidation with Ti has been proven effective in reducing corrosion current density [36][37]. Advancements in surface-engineering techniques and nanotechnology present the potential for improved prosthetic devices featuring selective bioactive surfaces, referred to as "intelligent surfaces". A major critique of current in vitro electrochemical characterization of medical implants is the absence of biological species within the simulated physiological media employed. The challenge for corrosion research lies in conducting electrochemical trials within media containing living cells and proteins. 316L unstained steel, along with titanium and other metallic materials, is commonly used for surgical implants. Unstained steel remains popular due to its relatively low cost, ease of manufacturing, and reasonable resistance against corrosion. However, it is susceptible to various forms of localized corrosion such as roughness and cranny corrosion, intergranular corrosion, and stress corrosion cracking [38].Stainless steel implants have dual purposes - they can serve as temporary implants for aiding in bone healing and permanent implants
for artificial joints. Temporary applications of stainless steel implants include plates, medullary nails, screws, pins, sutures, steel toggles, and webs used in fracture repairs [39]. While developed countries do not commonly use stainless steel as a permanent implant, it remains the most popular choice in emerging countries [40]. The susceptibility of stainless steel to different types of corrosion depends on environmental factors, chemical composition, and metallurgical fabrication. Various studies have been conducted to examine the effects of different anions present in the surrounding environment on the corrosion of stainless steel. For instance, Zuo et al discovered that chloride solutions containing OH-, NO3-, SO42-, ClO4-, and acetate ions can suppress roughness. Increasing the chromium content significantly improves resistance against localized passivation dislocation. There are several methods available for measuring and predicting the corrosion behavior of medical implant alloys that simulate real-life conditions. One such method is Electrochemical Impedance Spectroscopy (EIS), which measures resistance and capacitance of an oxide and models its behavior using a circuit to predict corrosion under varying conditions.The Potentiodynamic test is a method that measures various properties of a metal, including corrosion potential, breakdown potential, repassivation potential, and corrosion current density. This is achieved by using high amplitude DC voltage scans. The hysteresis area in the current vs. voltage curves can indicate how susceptible the metal is to corrosion.
Linear polarisation tests, on the other hand, involve applying a voltage slightly above and below the corrosion potential to predict the corrosion current based on the slope of the voltage vs. current curve.
A passivated metal surface with a stable oxide layer enhances its resistance to corrosion and inertness in physiological conditions. It is possible to
improve this passivity by modifying the thickness, morphology, or chemical composition of the surface oxide layer through different treatments. For instance, passivating 316L stainless steel can be achieved thermally, electrochemically or through treatment with nitric acid.
Our research aligns with previous studies in demonstrating that the behavior of steel depends on its surface treatment. When exposed to Ringer solution, it was observed that azotic acid passivated steel showed lower corrosion currents compared to electrochemically passivated steel and untreated steel.
Interestingly, we found that the corrosion potency of surface treated unstained steel was higher than that of untreated 316L stainless steel.The intervention on the surface of unstained 316L stainless steel improved not only its biocompatibility as an implant but also resulted in improved corrosion behavior overall.Various attempts have been made to modify the surface of metal implants with ceramic coatings in order to decrease corrosion rates and prevent the release of metallic ions or corrosion products. Research has demonstrated that applying ceramic coatings to metal implants can improve corrosion resistance, wear resistance, and bioreactivity, all of which are advantageous for medical devices. It is clear that continuous research and development of new technologies to enhance the performance of current SS 316L implants is extremely important.
Titanium has been a popular choice for implants because it has a thin native passive layer that provides excellent corrosion resistance. However, Titanium (Ti) has a high Young's modulus of 105 GPa and low bioactivity, both of which can result in inefficient implant development or implant failure. Many researchers have tried various surface treatments such as anodisation [47,49], ion nidation [48], chemical and thermal treatment [50-52], mechanical and chemical treatment [53], sol-gel deposition [54],
etc., in order to improve Ti biocompatibility.
The need for better implant materials prompted the creation of Ti metals with exceptional properties. Additionally, numerous Ti metals have been developed to meet key requirements for an ideal implant material.The requirements for implant materials include low density, a Young's modulus similar to bone, resistance to load-bearing, wear, fatigue, and tensile stress, high resistance to corrosive attacks from aggressive ions in human fluids (especially chloride ions), good biocompatibility, bioactivity, osseointegration, and osteoactivity. Recently, the properties of Titanium (Ti) as an implant material have been enhanced by incorporating binary, triple, quadruple, and multi-component metals. This section focuses on binary Ti metals that consist solely of non-toxic and non-allergic elements such as Niobium (Nb), Tantalum (Ta), Molybdenum (Mo), Gold (Au), Silver (Ag), Platinum (Pt), and Palladium (Pd). Ti-Nb metals are particularly notable due to the excellent mechanical properties and high corrosion resistance provided by niobium as a phase stabilizer component [55]. Research conducted by Wang et al. resulted in the development of Ti-22Nb metal while Lopes et al. studied Ti-33Nb metal [56][57]. Both binary alloys demonstrated higher corrosion resistance compared to pure Ti or other commercially available metals. However, their biocompatibility is yet to be determined. The Young's modulus of Ti-xNb alloys ranged from 72 to 62 GPa with a minimum value of 62 GPa observed for Ti-42Nb metal. Tantalum exhibits resistance against most acids except concentrated hydrofluoric acid. Investigation into Ti-Ta binary metals including Ti-13Ta and various compositions of Ti-xTa alloys (x = 30, 40 ,50 ,60) focused on evaluating their behavior in artificial saliva with different pH levels and fluoride content. These alloys exhibited superior performance when compared to
Ti-6Al-4V metal [58].Ti-Mo metals acquired a typical equiaxed grain microstructure and demonstrated exceptional corrosion resistance to aggressive ions from physiological fluid after undergoing tempering, slaking, cold peal, and recrystallization. In accordance with a study conducted by F. Rosalbino et al., Ti-1M alloys (Ag, Au, Pd, Pt) comprising of non-toxic elements exhibited superior mechanical properties and corrosion resistance compared to Ti and Ti-6Al-4V metal. In an environment containing fluoride, the stability of the inactive oxide layers of these metals increased which led to a reduction in surface activity. This was attributed to the integration of noble metal cations into the lattice structure of TiO2 which also enhanced its disintegration resistance. Furthermore, this article will discuss Ti-based ternary metals incorporating non-toxic elements like Nb, Zr, Ta, and Sn. Among these metals, extensive research has been carried out on Ti-13Nb-13Zr which possesses a modulus of 79 GPa and showcases high levels of corrosion and wear resistance in human biofluids while maintaining good biocompatibility. The osseointegration of this metal can be further improved by depositing apatite or hydroxyapatite on its surface; however, it is important to note that its high modulus may result in long-term complications under specific stresses. Additionally studied after heat treatment is the Ti-15Zr-4Nb metal whereby the composition analysis revealed that in its untreated state it possesses an inactive film composed of Ti2O3 oxide whereas in its heat-treated form the oxide layer consists primarily of rutile-form TiO2.The corrosion resistance of Ti-13Nb-13Zr metal was found to be lower than that of Ti-6Al-7Nb metal. Ti-xNb-13Zr metals, where x = 5, 13, or 20, have potential as implant materials due to their improved biomechanical compatibility and corrosion resistance compared
to Ti and Ti-6Al-7Nb when exposed to Ringer's solution. Different compositions of Ti-35Nb-xZr metals (x = 3, 5, 7, 10, or 15) were treated with anodal oxidization to create nanotubular oxide beds. These beds were then coated with hydroxyapatite using the magnetron sputtering method. The resulting thin film-coated nanotubular Ti-35Nb-xZr metal exhibited good corrosion resistance in a 0.9% NaCl solution [78]. When Sn was added to the Ti-Nb binary metals, it reduced the Young's modulus of the resulting Ti-Nb-Sn alloys. This decrease was attributed to Sn suppressing or slowing down phase transitions and ultimately leading to a Young's modulus of 40 GPa [58]. A thermo-mechanical process was used on a Ti-25Ta-Zr alloy with an ? + ? structure to optimize its strength-to-elastic modulus balance. This processing resulted in mechanical properties and a Young's modulus of 55 GPa. Additionally, the treated metal showed improved electrochemical behavior and mechanical properties due to the positive effects of the applied treatment [79].The metal surface underwent anodal oxidization [80], resulting in the formation of an oxide bed with controllable roughness, increased hydrophilicity, and excellent corrosion resistance. Additionally, we will examine quaternary Ti metals consisting only of biocompatible metals including Ti, Nb, Zr, and Ta. Researchers from Japan obtained and studied a cytotoxic-element-free Ti-15Zr-4Nb-4Ta metal with I± + I? construction [81-85]. This particular metal has a Young's modulus of 97 GPa and demonstrates a favorable balance between strength and ductileness. Moreover, it exhibits remarkable corrosion resistance and biocompatibility, making it suitable for implant applications. The metal also showcases high apatite-forming ability even in NaOH solution, CaCl2 solution, heat treatment, and water treatment [85,86]. Furthermore, this ability persists even in humid environments
while maintaining good scratch resistance after treatment. Another alloy known as Ti-29Nb-13Ta-4.6Zr [87,88] possesses a modulus of 60 GPa along with strong corrosion resistance in biofluids due to its protective oxides such as TiO2, Ta2O5,Nb2O5,and ZrO2.However,this alloy is considered bioinert as it cannot directly bond with living bone. Nevertheless,surface treatments like heat treatment[89]and heat treatment combined with calcium phosphate invert glass-ceramic[90]can enhance its bioactivity.Finally,the Ti-34Nb-9Zr-8Ta metal[91]has a I? microstructure alongside a relatively low elastic modulus of 89 GPa.Aging treatment can decrease its hardness and Young's modulus.Finally, the Ti-13Mo-7Zr-3Fe metal [91] undergoes composition and heat treatments to ensure a homogeneous I? structure with a Young's modulus of 89 GPa and good hardness. The Ti-35Nb-7Zr-5Ta metal [92,93], obtained through pulverization metallurgy, displays evenly distributed refined precipitates after aging intervention and exhibits high biocompatibility with a low Young's modulus, making it a promising candidate for implant materials.
1.3.3.Need for a new generation of durable implants
Implant metals must meet several requirements for long-term usage (20 years or more). They should have a low density and Young's modulus to promote bone bonding without causing bone reabsorption. Additionally, they should have high mechanical strength, fatigue resistance, and wear resistance [2,94] to prevent the release of ions, compounds or particles into surrounding tissues. Implant metals must also consist only of non-toxic and non-allergic elements to ensure good biocompatibility [1,95,96]. Furthermore, they should exhibit excellent long-term corrosion resistance to prevent the accumulation of ions and corrosion products in adjacent tissues. Over time, such accumulation can lead to significant local changes in biofluid pH and composition which may create gradients and accelerate corrosion in certain areas of the implant [97,98].Currently, the available implant metals
do not fully meet the requirements for durable implants. Therefore, researchers are focusing on developing a new generation of implant metals that only consist of biocompatible elements such as Titanium (Ti), Niobium (Nb), Zirconium (Zr), Tantalum (Ta), and others. Historical data has shown that the release of metallic ions into bodily fluids should not exceed 0.3 mg/L [84]. Ti, Nb, Ta, and Zr are particularly able to fulfill this requirement. Their biocompatibility decreases in the following order: Nb > Ta > Ti > Zr [95,96]. Additionally, these metals have high corrosion resistance due to protective oxides like ZrO2, Nb2O5, and Ta2O5 which strengthen the formation of a passive TiO2 film on their surfaces [99-101]. Nb is known for stabilizing the structure of I? and acts as a highly passivating metal [100]. Zr is an isomorphous metal soluble in both i??and I? Ti which limits corrosion at the i??stage and enhances corrosion resistance [63,102]. Although Ta exhibits superior corrosion resistance it has limited mechanical resistance [103,104]. Consequently, there is a demand for a new generation of durable implants that can meet various long-term usage demands including excellent biocompatibility, corrosion resistance comparable to bone's mechanical properties non-toxicity and non-allergic effects high fatigue wear resistance etc.However, there are only a few new metals that meet these requirements and there is limited information about how they react in physiological fluids.
1.3.4.Anticorrosive performances of some novel Ti-based metals
Three new quaternary Ti-Nb-Zr-...
- Organic Chemistry essays
- Acid essays
- Calcium essays
- Chemical Bond essays
- Chemical Reaction essays
- Chromatography essays
- Ethanol essays
- Hydrogen essays
- Periodic Table essays
- Titration essays
- Chemical reactions essays
- Osmosis essays
- Carbohydrate essays
- Carbon essays
- Ph essays
- Diffusion essays
- Copper essays
- Salt essays
- Concentration essays
- Sodium essays
- Distillation essays
- Amylase essays
- Magnesium essays
- Acid Rain essays
- Steel essays
- Agriculture essays
- Albert einstein essays
- Animals essays
- Archaeology essays
- Bear essays
- Biology essays
- Birds essays
- Butterfly essays
- Cat essays
- Charles Darwin essays
- Chemistry essays
- Dinosaur essays
- Discovery essays
- Dolphin essays
- Elephant essays
- Eli Whitney essays
- Environmental Science essays
- Evolution essays
- Fish essays
- Genetics essays
- Horse essays
- Human Evolution essays
- Isaac Newton essays
- Journal essays
- Linguistics essays