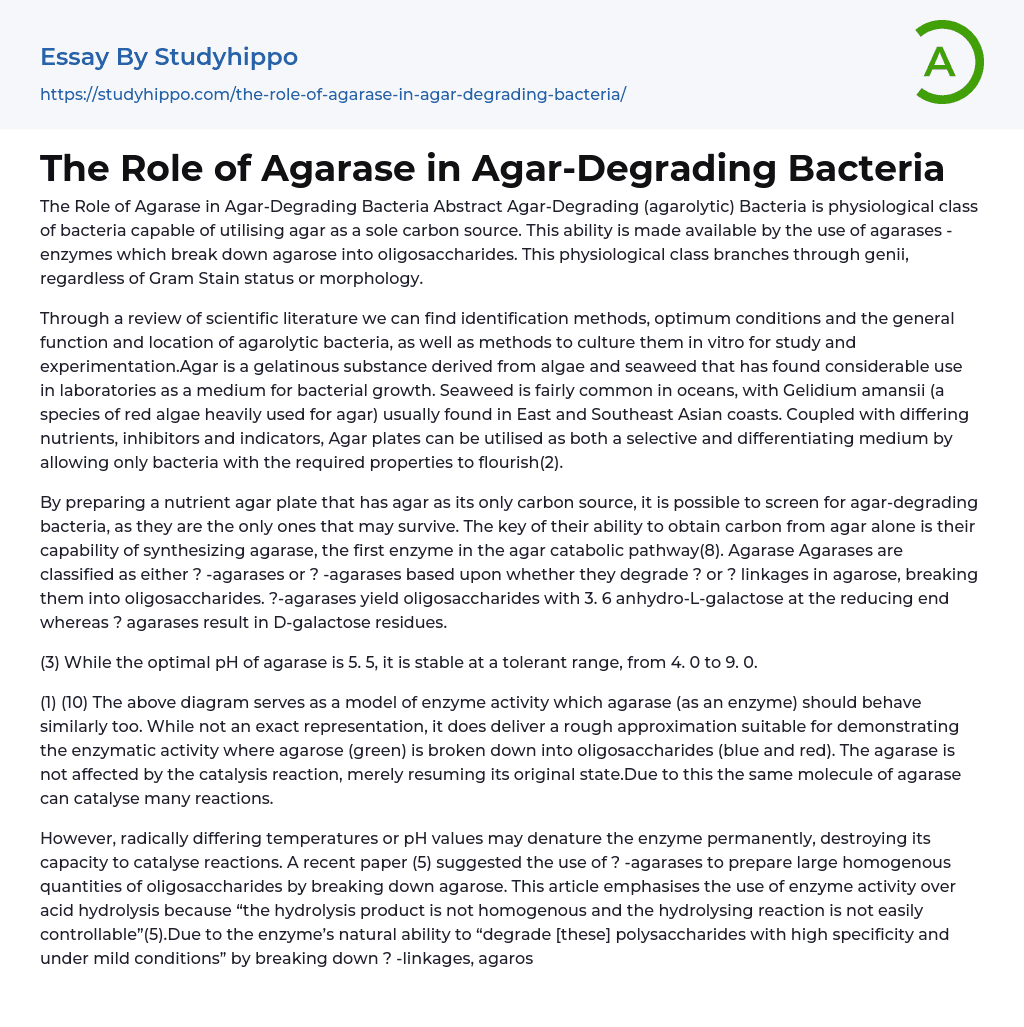
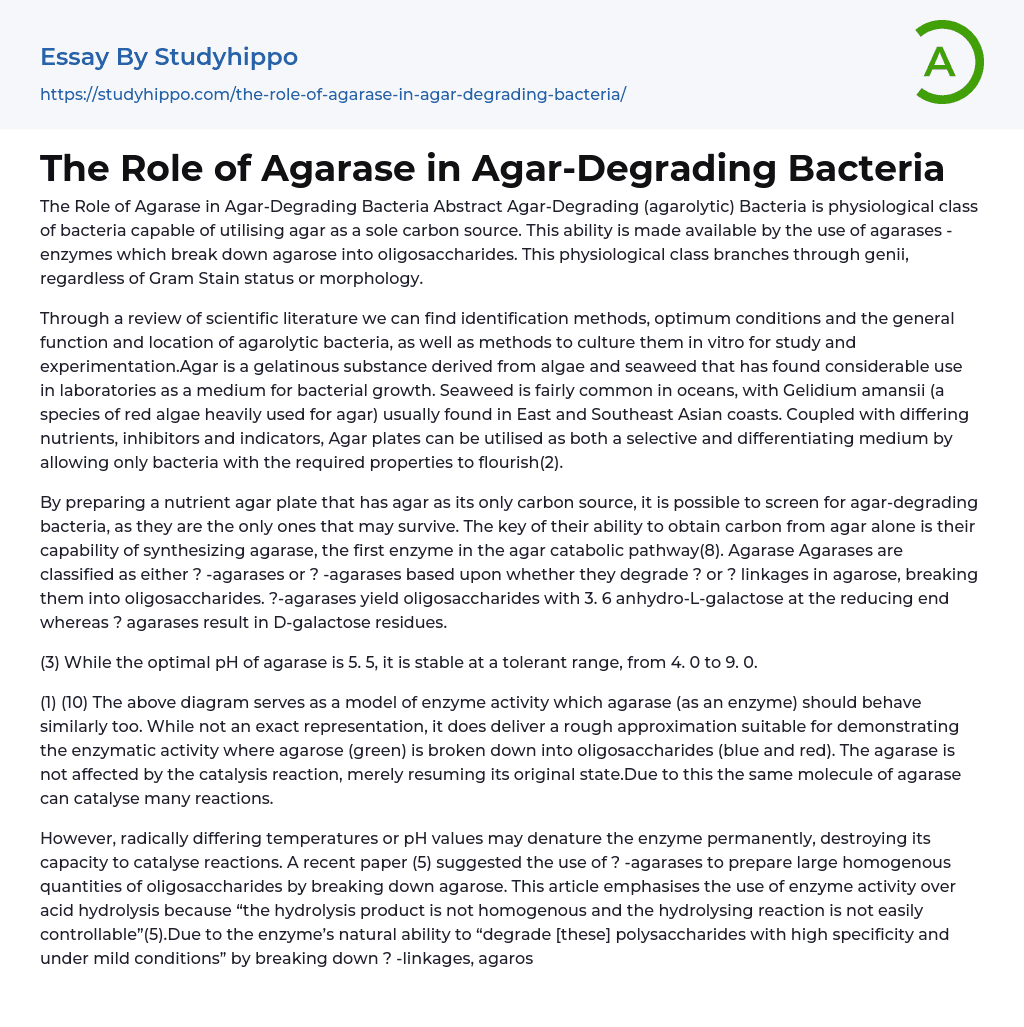
The Role of Agarase in Agar-Degrading Bacteria Essay Example
The Role of Agarase in Agar-Degrading Bacteria Abstract Agar-Degrading (agarolytic) Bacteria is physiological class of bacteria capable of utilising agar as a sole carbon source. This ability is made available by the use of agarases - enzymes which break down agarose into oligosaccharides. This physiological class branches through genii, regardless of Gram Stain status or morphology.
Through a review of scientific literature we can find identification methods, optimum conditions and the general function and location of agarolytic bacteria, as well as methods to culture them in vitro for study and experimentation.Agar is a gelatinous substance derived from algae and seaweed that has found considerable use in laboratories as a medium for bacterial growth. Seaweed is fairly common in oceans, with Gelidium amansii (a species of red algae heavily used for agar) usually found in East and Sou
...theast Asian coasts. Coupled with differing nutrients, inhibitors and indicators, Agar plates can be utilised as both a selective and differentiating medium by allowing only bacteria with the required properties to flourish(2).
By preparing a nutrient agar plate that has agar as its only carbon source, it is possible to screen for agar-degrading bacteria, as they are the only ones that may survive. The key of their ability to obtain carbon from agar alone is their capability of synthesizing agarase, the first enzyme in the agar catabolic pathway(8). Agarase Agarases are classified as either ? -agarases or ? -agarases based upon whether they degrade ? or ? linkages in agarose, breaking them into oligosaccharides. ?-agarases yield oligosaccharides with 3. 6 anhydro-L-galactose at the reducing end whereas ? agarases result in D-galactose residues.
(3) While the optimal pH of agarase is
5. 5, it is stable at a tolerant range, from 4. 0 to 9. 0.
(1) (10) The above diagram serves as a model of enzyme activity which agarase (as an enzyme) should behave similarly too. While not an exact representation, it does deliver a rough approximation suitable for demonstrating the enzymatic activity where agarose (green) is broken down into oligosaccharides (blue and red). The agarase is not affected by the catalysis reaction, merely resuming its original state.Due to this the same molecule of agarase can catalyse many reactions.
However, radically differing temperatures or pH values may denature the enzyme permanently, destroying its capacity to catalyse reactions. A recent paper (5) suggested the use of ? -agarases to prepare large homogenous quantities of oligosaccharides by breaking down agarose. This article emphasises the use of enzyme activity over acid hydrolysis because “the hydrolysis product is not homogenous and the hydrolysing reaction is not easily controllable”(5).Due to the enzyme’s natural ability to “degrade [these] polysaccharides with high specificity and under mild conditions” by breaking down ? -linkages, agarose is degraded both efficiently and reliably. This technique relies on the production of extracellular agarase from strains of E.
coli modified to carry AgaA or AgaB (different types of ? -agarases) encoding genes and is the same way the process is carried out in nature, with bacteria releasing agarase to break down agar outside of the cell gradually until it is in a form suitable to be utilized by the bacteria.It can be seen that agarase defines agar-degrading bacteria, as its namesake function is not possible without it. Environment and Nutrients As could be expected, many species of agar-degraders
are marine micro-organisms – an adaptation to their environment which would be wasted in the majority of other micro-organisms such as those that exist on land (although there are such examples, including a species of Paenibacillus in the Rhizosphere of Spinach (4)). From species within genus Vibrio (1) toAlteromonas (6), the presence of agarase allows agar-degrading bacteria an abundant food source in the ocean. The same research by Leon et al also demonstrates that glucose can inhibit extracellular agarase secretion (but not transcription), causing it to degrade within the cell and thus limit growth of the bacteria.
In addition, a study of the effects of phosphate limitation on agarase shows that limiting phosphate increases both intracellular agarase production and extracellular secretion, whereas a magnesium limitation does not (9).This further highlights the niche which this class of bacteria usually occupies, as the concentration of glucose or phosphate in the ocean is very low while magnesium concentration is generally much higher, suiting the agar-degrading bacteria’s agarase production; there is simply no need to use glucose in the ocean, so many organisms can’t. In Vitro Culturing Knowing the conditions agar-degrading bacteria accommodate in situ, it is possible to approximate similar conditions in vitro. Leon et al achieved this by spreading samples of sea water on agar plates containing 0. 5% casein hydrolysate, 0. 05% yeast extract, 2.
5% NaCl, 0. 06% NaH2PO4, 0. 5% MgSO4, 0. 002% FeSO4•7 H20, 0.
01% CaCl2 and 1. 5% Agar with a pH of 7. 25 and incubating them for 48 hours at 25°C(6). After 48 hours, single colonies that had made small pits in the agar (thus indicating the presence of
agarase) were inoculated onto new plates with the same conditions as above. The aim of this selective and differential process is to ensure a pure culture, as non-agarolytic bacteria should not be able to survive the first plate, let alone a second one.
This inoculation technique is the same standard one used in microbiological laboratories such as those at the University of New South Wales. Note that the chosen medium contains very little phosphate and no other carbon source such as glucose, yet it does contain a fair amount of magnesium. This serves to simultaneously optimise it for agarolytic bacteria and inhibit the growth of other physiological classes by removing the ability for other organisms to grow and simulating the standard conditions encountered by most of the later.Also, the incubation temperature should be taken into account. 25°C is well above the temperatures usually experienced by agar-degrading bacteria as they exist in oceans, which are generally cold.
This may affect the culture yield and should be examined. To identify the bacteria, the researchers consulted Bergey’s Manual of Systematic Bacteriology and ran tests involving staining, morphology, motility and oxidation as prescribed by texts cited in the article (6).A statement of characteristics included in the article claims that Alteromonas sp. Strain C-1 was characterised by “Gram negative rods, 0.
7 by 2. 3? m; obligately aerobic; motile by means of a polar flagellum; catalase and oxidase positive; indol and arginine dihydrolase negative. ” Included below is a table showing other biochemical tests carried out: (6) It is through these tests that the bacterium was classified as an Alteromonas species.The morphology and Gram staining help to identify a broader taxonomy
by comparison (eliminates cocci, spirochetes, etc and bacteria with no outer membrane), while catalase and oxidase tests indicate aerobic respiration (requiring oxygen) and narrow the field even further. The motility test indicates that the bacteria are capable of locomotion by rotating a whip like tail. Though these tests are critical for deciding taxonomic rankings such as genus and species, there is only one test needed to decide whether the bacteria in question is an agar-degrading one: Growth in a medium with agar as the sole carbon source.
This can be determined by the effect of the bacteria on the medium. If there are pits beneath colonies on the agar, this indicates that the agar substrate has been catalysed by secreted agarase and the colony secreting it is indeed agarolytic, because no other physiological class will affect the agar this way. Diversity of Micro-Organisms Though all genii of agar-degrading bacteria are capable of degrading agar, they may still differ from one another to varying degrees. Even in the use of either ? - or ? agarases – the simple mechanisms by which they degrade agar(3) – we see variation.
Naturally, we see differences across the genii – Streptomyces coelicolor and Pseudomonas atlantica are both agarolytic, but the former stains Gram Positive while the latter stains Gram Negative(7). This is a very important compositional difference, yet they both retain a similar function. This can be largely explained by the concept of “convergent evolution”, where unrelated organisms will form similar features in response to the same need (in this case, the need to utilise agar).The efficiency of agarase can be attested to by the large variety of bacteria that
survive through its capabilities. Through all types of agarolytic bacteria, agarase remains the constant, whether ? or ?. As an enzyme created through need, it defines an entire physiological category and enables a function that wouldn’t be efficiently possible otherwise.
Growing both in oceans and 25°C agar plates, agar-degrading bacteria show some compatibility with change and stress, producing agarase until conditions (such as an increase in glucose or drastic change of pH) deem it inappropriate.Through careful research, specific requirements for different strains can be discovered and utilised to culture the bacteria for purposes ranging from research to industrial applications. Future usage could include Jingbao et al’s ideas of replacing acid hydrolysis - lowering required energy by using the enzymes of bacteria literally designed for the job – or even applying agar-degrading bacteria and agarase to “algal bloom”, using natural means to clean up ecological issues. References 1. Aoki, T. , T.
Araki, and M. Kitamikado. 1990. Purification and characterization of a novel ? agarase from Vibrio sp. AP-2 European Journal of Biochemistry 187:461-465.
2. Atlas, R. M. 2004. Handbook of Microbiological Media, 3rd ed, vol.
CRC Press. 3. Hassairi, I. , R. Ben Amar, M. Nonus, and B.
B. Gupta. 2001. Production and separation of ? -agarase from Altermonas agarlyticus strain GJ1B. Bioresource Technology 79:47-51. 4.
Hozoda, A. , M. Sakai, and S. Kanazawa. 2003.
Isolation and characterization of Agar-degrading Paenibacillus spp. Associated with the Rhizosphere of Spinach Bioscience, Biotechnology, Biochemistry 67:1048-1055. . Jingbao, L.
, H. Feng, L. Xinzhi, F. Xiaoyan, M.
Cuiping, C. Yan, and Y. Wengong. 2007. A simple method of preparing diverse neoagaro-oligosaccharides with ? -agarase.
Carbohydrate Research 342:1030-1033. 6. Leon,
O. , L. Quintana, G. Peruzzo, and J.
C. Slebe. 1992. Purification and Properties of an Extracellular Agarase from Alteromonas sp. Strain C-1. Purification and properties of Extracellular Agarase from Alteromonas sp.
Strain C-1 58:4060-4063. 7. Ndip, R. N.
, E. C. Beeching, L. M. Ndip, W.
F. Mbacham, and V. P. Titanji. 2007.Molecular characterisation of Pseudomonas aeruginosa recovered in the Buea health district of Cameroon: implications for nosocomial spread.
West African Journal of Medicine 26:191-195. 8. Parro, V. , and R. P.
Mellado. 1994. Effect of glucose on agarase overproduction in Streptomyces. Gene 145:49-55. 9. Parro, V.
, R. P. Mellado, and C. R. Harwood.
1998. Effects of phosphate limitation on agarase production by Streptomyces lividans TK21. FEMS Microbiology Letters 158:107-113. 10. Vickers, T.
2006. Induced Fit Diagram. Wikipedia Foundation.