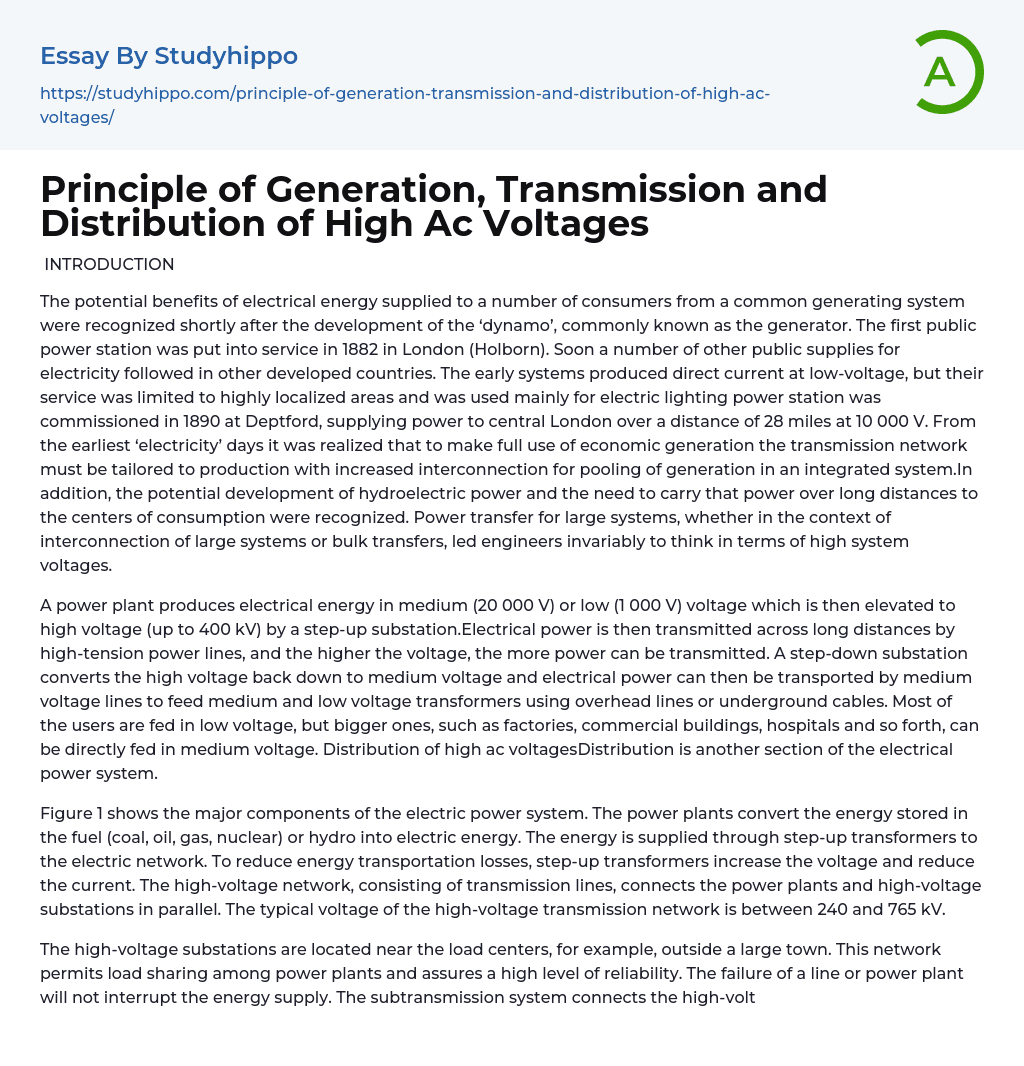
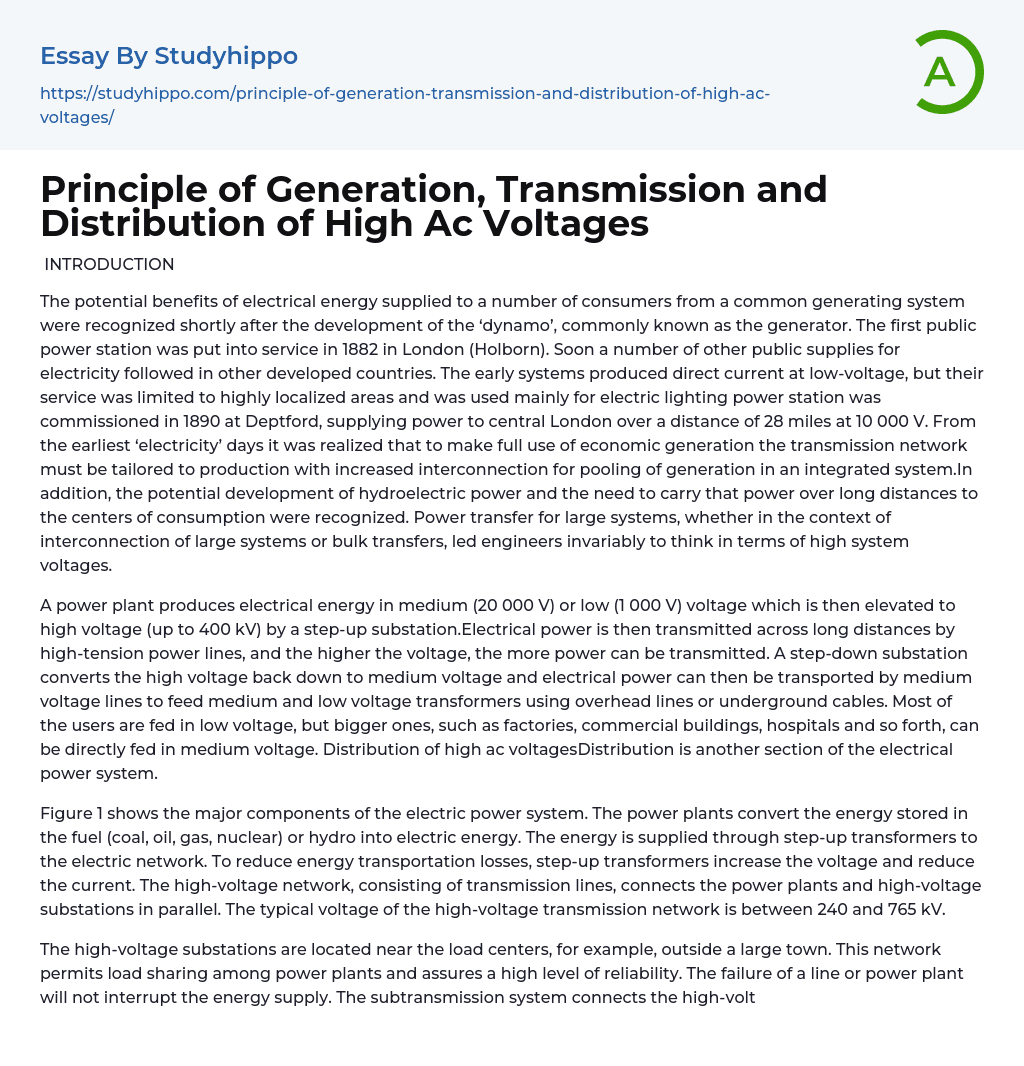
Principle of Generation, Transmission and Distribution of High Ac Voltages Essay Example
INTRODUCTION
Shortly after the creation of the generator, commonly referred to as the 'dynamo', the benefits of supplying electrical energy to multiple users from a shared power generation system were recognized.
In 1882, the first public power station began operating in London (Holborn), and other countries quickly followed suit by establishing their own electricity supply systems. Initially, these systems produced low-voltage direct current, mainly for electric lighting in localized areas. However, a power station was commissioned in Deptford in 1890 to provide power to central London over a distance of 28 miles at 10,000 V. This highlighted the importance of an integrated transmission network during the early days of electricity to maximize efficient generation.
Increased interconnection enabled the merging of generation and facilitated long-distance transmission of hydroelectric power to consumption centers. To achieve this, power plants
generate electrical energy at medium (20,000 V) or low (1,000 V) voltage and then increase it through a step-up substation to high voltage (up to 400 kV). High-tension power lines are used to transmit this electrical power across long distances, with higher voltages enabling greater transfer capabilities.
A step-down substation is responsible for converting high voltage to medium voltage so that electrical power can be transmitted through medium voltage lines. This power is then used to supply medium and low voltage transformers, either through overhead lines or underground cables. While most users are supplied with low voltage power, larger facilities like factories, commercial buildings, and hospitals can receive power directly through medium voltage. Additionally, the distribution of high ac voltages is another important aspect of the electrical power system. Figure 1 illustrates the main components of this system, including power plants that conver
various fuel sources (coal, oil, gas, nuclear) or hydro energy into electric energy.
The energy is transported to the electric network through step-up transformers that increase voltage and decrease current. This helps minimize energy loss during transportation. The high-voltage transmission network, which consists of transmission lines, connects power plants and high-voltage substations in parallel. Typically operating at voltages between 240 and 765 kV, these substations are strategically positioned near load centers, such as outside major cities.
The network enables power plants to share the load and ensures a high level of reliability, so that a failure in a power plant or line will not disrupt the energy supply. The subtransmission system connects high-voltage substations to distribution substations located directly in load centers, such as urban areas which have distribution stations around 5 to 10 miles apart. The subtransmission system typically operates at voltages between 138 and 69 kV. In areas with high load density, the subtransmission system employs a network configuration resembling the high-voltage network.
In medium and low load density areas, the loop or radial connection is used. Figure 65.1 shows a typical radial connection. The distribution system has two parts, primary and secondary. The primary distribution system consists of overhead lines or underground cables, which are called feeders. The feeders run along the streets and supply the distribution transformers that step the voltage down to the secondary level (120–480 V). The secondary distribution system contains overhead lines or underground cables supplying the consumers directly (houses, light industry, shops, etc).
) by single- or three-phase power. Separate, dedicated primary feeders supply industrial customers requiring several megawatts of power. The sub transmission system directly supplies large factories consuming
over 50 MW.
The most frequently used voltages and wiring in the primary distribution system are listed in Table 1. Primary distribution, in low load density areas, is a radial system. This is economical but yields low reliability. In large cities, where the load density is very high, a primary cable network is used.
The distribution substations are linked through feeders, which can be either lines or cables. Short-circuit protection is provided by circuit breakers (CBs) installed at both ends of the feeder. The connection to the loads is made directly through fuses. This linking process resembles the one-line diagram portrayed in Fig. in the high-voltage network.
1. The network's high cost limits its application. However, a more cost-effective and reliable alternative is the loop connection, which involves powering the main feeder from two independent distribution substations. These substations have four outgoing main feeders, each supplying a different load area and protected by a reclosing circuit breaker (CB). The main feeders, consisting of three-phase four-wire systems, then supply single-phase lateral feeders.
A recloser and a sectionalizing switch are used to divide the main feeder into two parts. To connect the feeder to the adjacent distribution substation, a normally open tie-switch is utilized. In case of a fault occurring between the circuit breaker (CB) and recloser, the reclosing CB is opened. However, after a few cycles, the CB is reclosed.
If the fault is not cleared, the opening and reclosing process is repeated twice. If the fault is still not cleared after the third reclosing, the circuit breaker (CB) remains open. Then, the sectionalizing switch opens and the tie-switch closes. This activates the feeder between the recloser and
the tie-switch from the neighboring feeder. Likewise, the fault between the recloser and tie-switch triggers the recloser to open and reclose three times.
If the fault is not cleared, the recloser remains open and separates the faulty part of the feeder. FIGURE 2 shows a radial primary distribution system, which is effective for overhead lines where temporary faults are often caused by lightning, wind, and metal balloons. In this system, a three-phase switched capacitor bank is installed two-thirds of the distance out on the feeder from the source and is rated two-thirds of the total average reactive load. The capacitor bank improves the power factor and reduces voltage drop at heavy loads. However, to avoid over voltages, the capacitor is switched off at light loads.
Some utilities use voltage regulators at the primary feeders. These voltage regulators are autotransformers, equipped with a secondary coil that has 32 taps. A switch is connected to the selected tap on the secondary coil, allowing the regulation of voltage in the line. However, there is a limitation to the lifetime of the switch used in the tap changer, which restricts the number of operations it can perform per day. To ensure equal phase loading, the lateral single-phase feeders are supplied from different phases. Furthermore, fuse cutouts are implemented to protect the lateral feeders.
These fuses are designed to work in conjunction with the fuses that protect the distribution transformers. If there is a fault in the distribution transformer, the transformer fuse will be the first one to melt. In case of a fault in the lateral feeder, the cutout fuse will operate before the recloser or circuit breaker opens permanently. The larger
loads on the three-phase line are safeguarded by circuit breakers or high-power fuses. In rural areas, most primary feeders use overhead lines with distribution transformers mounted on poles. The capacitor banks, reclosing switches, and sectionalizing switches are also pole-mounted.
The installation costs of overhead lines may be lower, but they lack aesthetics. In urban areas, an underground cable system is employed, where switchgear and transformers are housed in underground vaults or ground-level cabinets. This underground system is unaffected by weather and is very dependable. However, the initial cost of an underground cable is much higher compared to an overhead line of the same capacity. This expense restricts the use of underground systems to high-density urban areas and housing developments, and flooding can pose challenges.
The secondary distribution system delivers electric energy to customers via distribution transformers and secondary cables. Table 2 displays the typical voltages and wiring arrangements. In residential areas, the single-phase three-wire 120/240-V radial system is commonly utilized, with lighting loads powered by the 120 V line and larger household appliances (such as air conditioners, ranges, ovens, and heating systems) connected to the 240-V lines. The choice between underground cables or overhead lines depends on the location. In certain situations, HVDC may be chosen due to technical considerations, serving as the only practical option for asynchronous interconnection.
When deciding between alternating current (ac) and high voltage direct current (HVDC) for long-distance power transmission, the choice depends on the overall costs. The total cost includes line costs such as conductors, insulators, and towers, as well as obtaining right-of-way (R-o-W). A DC line with two conductors can transport a similar amount of power to a three-phase AC line
with equivalent-sized conductors. However, DC towers with only two conductors are simpler and less expensive than three-phase AC towers. As a result, the per-mile costs for both the line and R-o-W are lower for a DC line. Additionally, power losses in a DC line are lower when transmitting the same amount of power compared to AC. Nevertheless, HVDC systems require converters at both ends of the line, resulting in higher terminal costs compared to AC.
Variation of total costs for AC and DC as a function of line length are depicted in Fig. [figure number]. Above a certain distance, the total costs of DC transmission option become lower than the AC transmission option. For overhead lines, this break-even distance falls within the range of 500 to 800 km, whereas for cables it is considerably shorter. For submarine cables, it is between 20 and 50 km, and for underground cables, it is twice that distance.
- Energy Development essays
- The elements essays
- Voltage essays
- Solar Energy essays
- Nuclear Energy essays
- Alternative Energy essays
- Teenagers essays
- Millennials essays
- Teenage Drinking essays
- Teenage Suicide essays
- Atom essays
- Big Bang Theory essays
- Density essays
- Electricity essays
- Energy essays
- Force essays
- Heat essays
- Light essays
- Motion essays
- Nuclear Power essays
- Physiology essays
- Sound essays
- Speed essays
- Temperature essays
- Thermodynamics essays
- Anthropology essays
- Audience essays
- Charity essays
- Cultural Competence essays
- Emile Durkheim essays
- Gender Roles essays
- Generation essays
- Globalization essays
- Interpersonal Relationship essays
- People essays
- Race essays
- Social Change essays
- Social Class essays
- Social Movement essays
- Social Science essays
- Social Status essays
- Social Stratification essays
- Society essays
- Sociological Imagination essays
- Sociological Perspective essays
- Sociological Theories essays
- Stereotypes essays
- Web Dubois essays
- Attitude essays
- Goals essays